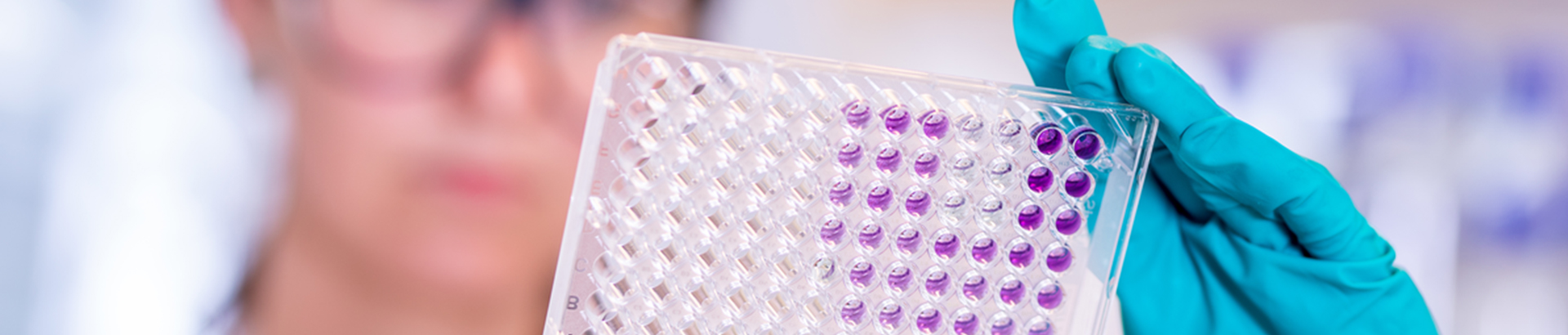
Research on Material Preparation
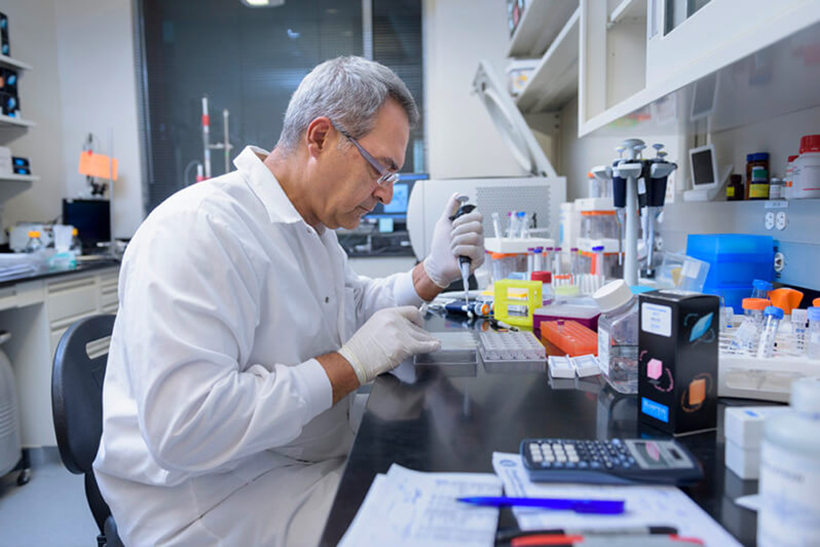
Polymer synthesis
Polymers are a type of substance composed of small repetitive chemical units. The synthetic blocks or repeating units in polymers are called monomers. Polymerization or polymer synthesis is a chemical reaction in which monomers are covalently bonded together to form a polymer structure. The length of a polymer chain is expressed by the number of repeating units in the chain, which is called the degree of polymerization (DP). The molecular weight of a polymer is the product of the repeated unit molecular weight and DP. The inherent basic characteristics of polymers mainly depend on their molecular weight, structure (straight or branched), and DP.
According to the types of chemical reactions involved, polymerization can be divided into two categories, namely condensation polymerization and addition polymerization. In condensation polymerization or stepwise growth polymerization, a condensation reaction occurs between two different bifunctional or trifunctional monomers, producing a polymer that eliminates small molecules (usually water) in the reaction. In addition polymerization or chain growth polymerization, polymer growth is achieved by adding monomers to the active sites at the end of the polymer chain and returning to the active sites after each growth step is completed. An initiator is required to produce an initiator substance with a reaction center. The reaction center can be a free radical, cation, anion, or organometallic complex.
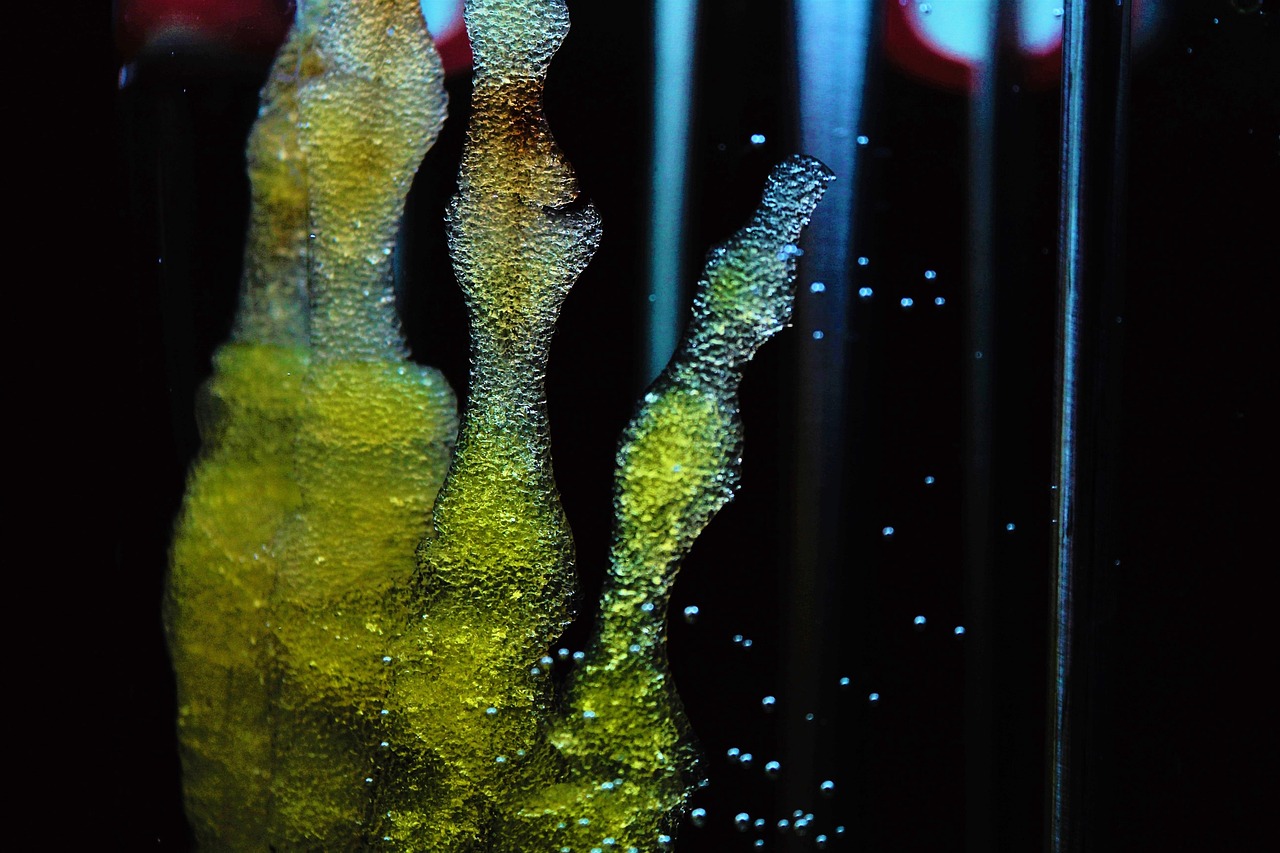
bioelectronics
Bioelectronics is an interdisciplinary field that combines biology and electronics, and can be used for diagnostic and therapeutic healthcare applications. By utilizing bioelectronic technologies that influence specific molecular processes in neural signal transduction, the regulatory activity of the nervous system can be monitored and controlled. Bioelectronic medicine deals with a series of diseases and obstacles, such as blindness, cardiovascular diseases, diabetes, inflammatory and neurodegenerative diseases and paralysis. Bioelectronic devices work by stimulating, regulating, or blocking specific electronic communication signals between the brain and functional organs of the body, thereby achieving personalized treatment. Common biomedical devices include pacemakers that regulate heart rate and robotic prosthetics that mimic the human environment. Biosensors such as blood glucose monitors can detect enzymes, pathogens, or toxic substances. Wearable bioelectronic devices can monitor vital signs, detect biomarkers, or capture epidermal energy. Advanced bioelectronic implants can work without wires or batteries, and according to different applications, they have the characteristics of minimal invasiveness, digestibility, and complete absorption.
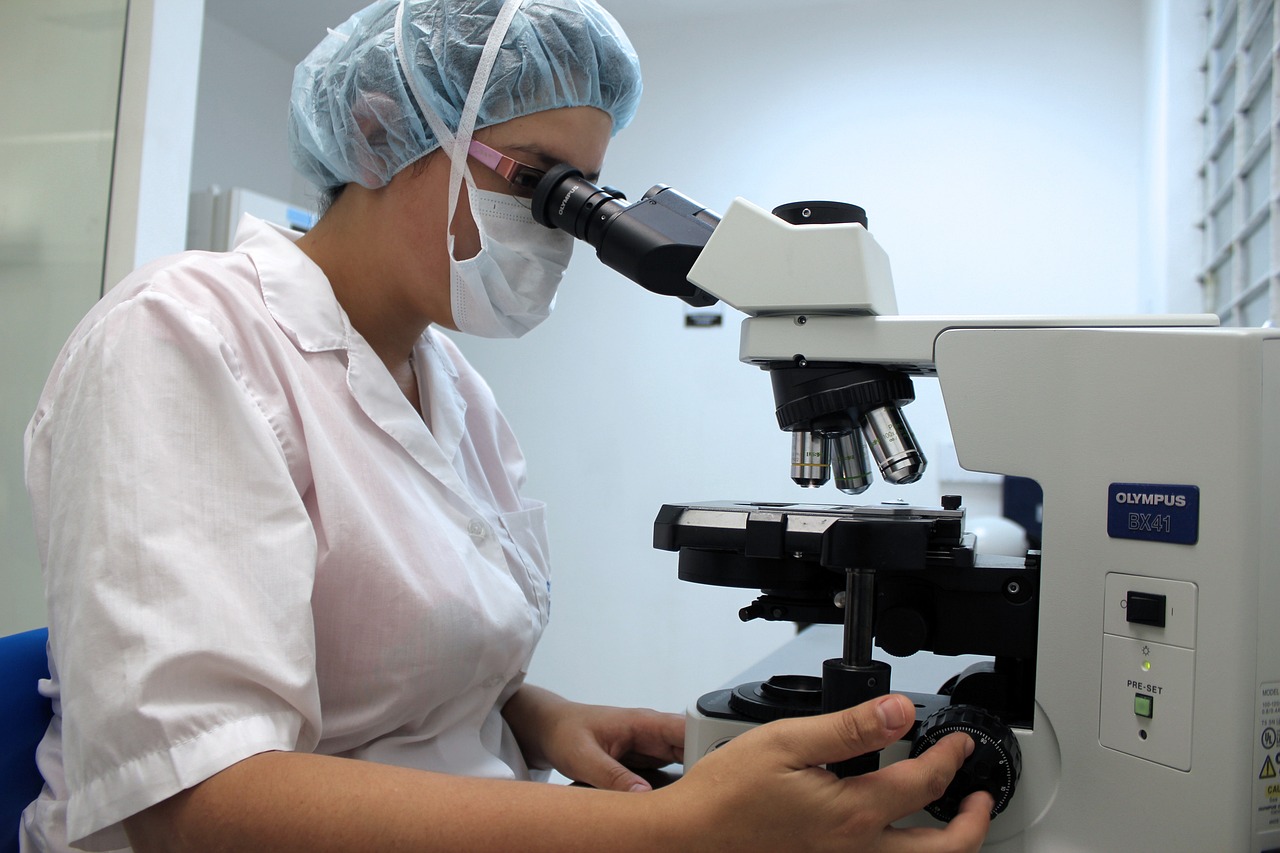
Synthesis of Nanoparticles and Microparticles
Micron particles and nanoparticles, as unique materials, have enormous potential for application in fields such as energy, healthcare, and the environment. Nanoparticles refer to particles with at least one physical dimension smaller than 100 nanometers. Micron particles refer to particles with a physical dimension ranging from 1 to 1000 micrometers. Although they have the same composition in macroscopic materials, the two types of particles have different optical, electrical, thermal, and magnetic properties due to size effects. Researchers have developed various methods for synthesizing two types of particles to better control their properties, shape, composition, and size distribution that are suitable for specific fields Usually, physical and chemical methods are used for synthesis. Physical methods synthesize particles by reducing the size of raw materials, which is known as a top-down approach. Usually, physical technologies such as ball milling, gas condensation, electrospray, lithography, and thermal decomposition are required. In many chemical methods, particles are usually synthesized by nucleation and growth of atomic or molecular precursors in liquid or gas phase chemical reactions, which is known as a bottom-up approach. Microemulsion method, hydrothermal method, microfluidic method, chemical vapor deposition, pyrolysis method and sol gel method are commonly used chemical synthesis methods. The nanostructures synthesized by chemical synthesis have fewer defects, allowing for more complex and uniform chemical composition. Moreover, due to their ease of expansion, the synthesized production cost is low and the speed is fast.
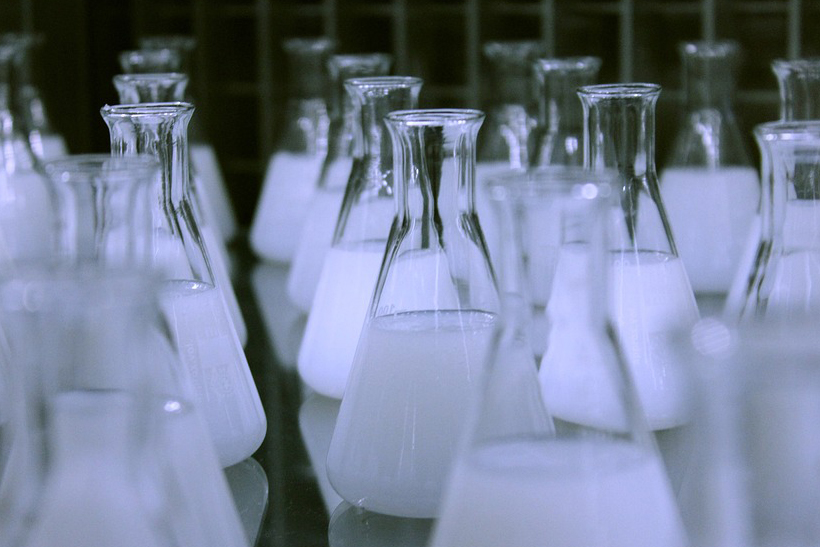
Solid State Synthesis
Solid state synthesis, also known as ceramic synthesis, is commonly used to initiate chemical reactions in solid starting materials, thereby forming new solids with regular structures. The final products include polycrystalline materials, monocrystalline materials, glass, and thin film materials widely used in energy and electronic applications. Under controlled temperature, fine metal compounds are combined with each other, then granulated and heated for a period of time. Some metal compounds (such as metal oxides or salts) require extreme conditions such as high temperature and high pressure to initiate reactions in the melt or rapidly condensing vapor phase. This process is often referred to as "shaking and baking" or "heating and striking" chemistry The reaction rate in solid-state synthesis is a particularly important characterization parameter. Due to the limited purification technology for the formed solid, the solid-state reaction must be complete. The reaction rate of solid-state reactions depends on the reaction conditions, including structural characteristics, shape and surface area of reactants, diffusion rate, and thermodynamic properties related to nucleation/reaction. The physical and chemical properties of the final material depend on the chemical precursors and preparation techniques.
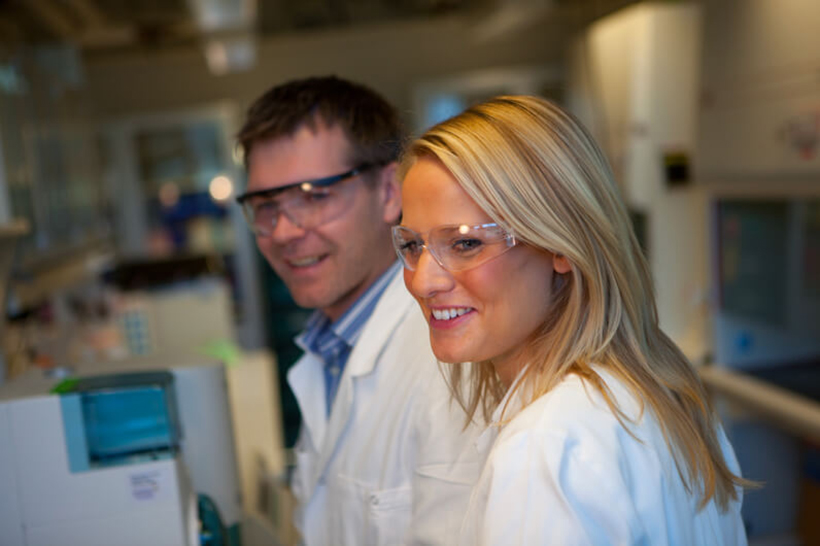
tissue engineering
Tissue engineering and regenerative medicine are interdisciplinary fields aimed at developing biological alternatives to repair, maintain, and improve tissue function or replace diseased or injured tissues. Hard tissues (such as bones and cartilage) and soft tissues (such as skin and cardiovascular valves) can both be constructed through tissue engineering. Cells, cytoskeleton, and growth stimulation signals are the three major elements of engineering tissue substitutes. Although tissue engineering culture involves in vitro tissue culture, regenerative medicine combines tissue engineering with other strategies, including cell therapy, gene therapy, and immune regulation, to induce in vivo tissue regeneration. Researchers have also developed biomimetic tissue constructs as in vitro models for drug screening and disease modeling.
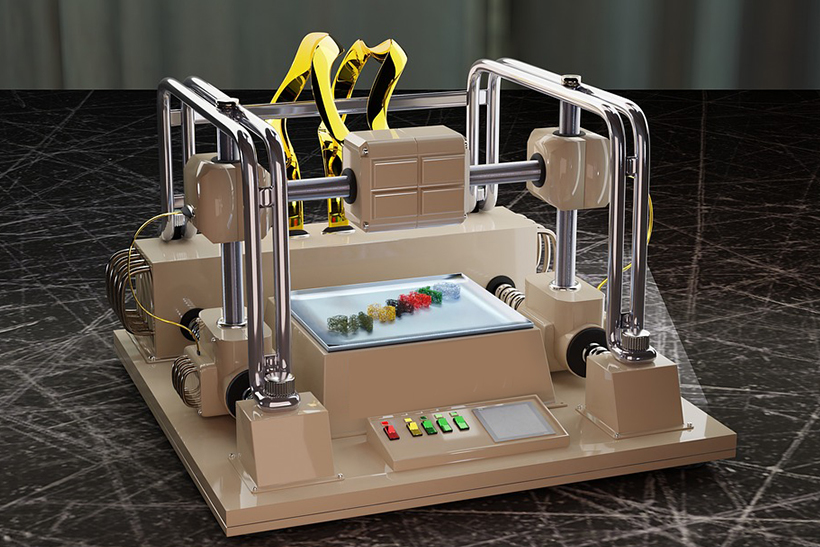
3D bioprinting
3D bioprinting is a process of additive manufacturing using biomaterials, live cells, and active biomolecules to create structures that simulate natural tissue characteristics. The main difference between biological printing and 3D printing is that living cells are added to non-toxic hydrogels to simulate the extracellular matrix environment and support cell adhesion, proliferation and differentiation after printing. The bioprinting process starts with 3D imaging to obtain precise tissue dimensions. Similar to conventional 3D printing, biological 3D printing also uses layer by layer instructions to create digital models, making it easier to create physical 3D objects. In order to optimize cell viability and ensure that the printing resolution is sufficient to evenly distribute cells, sterile printing conditions are required. According to different applications, biological materials that support cell growth (such as alginate, collagen, gelatin, or hyaluronic acid) are combined with living cells to form bio inks. Utilize highly controlled layer by layer methods to deposit bio ink through extrusion, inkjet, or laser 3D printing techniques. These 3D organizational structures are solidified through UV, chemical stimulation, or heating to obtain a stable growth environment.
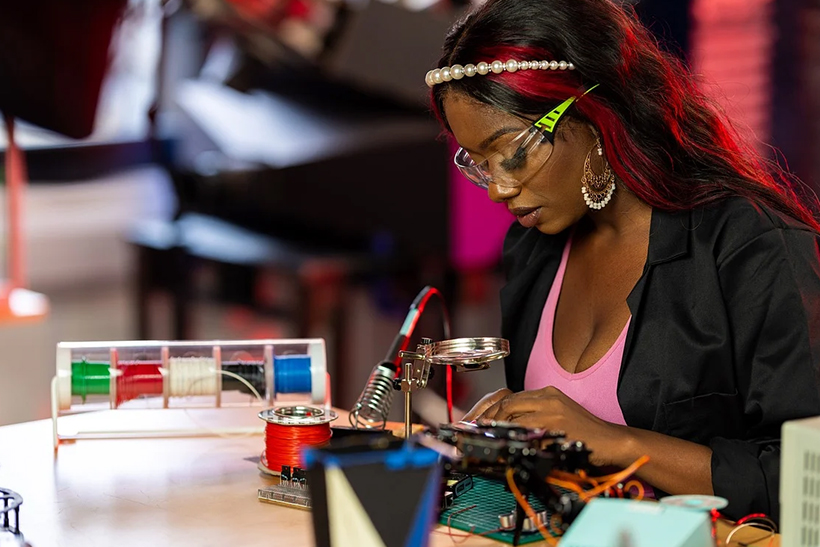
Organic electronics
Organic electronics uses organic polymers or small molecules to prepare electronic components for various new application fields. Compared with traditional silicon-based inorganic materials, organic electronic materials are lighter, more flexible, and cheaper. The production, use, and disposal of organic electronic devices also save more energy and resources. The preparation of small molecule organic electronic devices usually requires vacuum deposition to transfer organic material thin films to the substrate surface. Organic electronic devices can be prepared using conductive polymers through low-cost solution processing methods. Organic semiconductor materials can be made into soluble substances and converted into ink, allowing electronic circuits to be printed directly onto large plastic films. These materials are very suitable for the easy to expand large-area roll to roll manufacturing process, which can achieve fast and low-cost production.